Wearable Technology in Medicine with John A. Rogers, PhD
John A. Rogers, PhD, has created a fleet of wireless, wearable devices that have the potential to change the way physicians collect data and treat patients, from NICU preemies to stroke patients in recovery.
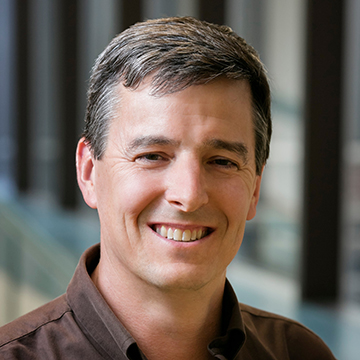
"We can do real-time biomarker analysis of sweat, we have devices that can measure ambient conditions, pollution levels, UV exposure levels and so on. It's a platform and we take it in different directions. Typically, identifying unmet clinical needs in close collaboration with folks at Feinberg."
— John A. Rogers, PhD
Professor of McCormick School of Engineering and Neurological Surgery
Episode Summary
Rogers' wearable devices can take measurements far beyond those made by an Apple Watch or FitBit.
John Rogers: "The electronics modules in those kinds of wearables are basically rigid, planar blocks of electronics. And it's great for a lot of things, but it rattles around in a loosely coupled fashion to the wrist or sometimes the chest, and the absence of that intimate interface really only allows you to make measurements of things like steps or activity level, a rough approximation of heart rate. But it can't allow you to capture electrocardiograms for example."
His wireless devices use new electronic materials and biosensors that are naturally compatible with the skin.
John Rogers: "What we're trying to do is reproduce electronic function in a platform that's reminiscent of a children's temporary tattoo. We feel that kind of technology represents the optimal way to integrate devices with the skin, in a way that's essentially imperceptible, because the technology adopts the physical characteristics of the skin itself. That provides a continuous intimate interface to the skin such that you can use the skin itself as a window into making all of these sort of clinical grade measurements of health status."
The devices send results to specially designed apps on smart phones and tablets, using the same kind of wireless capability present in all smartphones.
John Rogers: "The devices themselves, they don't have switches or displays or there anything else. Everything is happening in a virtual mode through, through an app typically running on a tablet or a smartphone....one thing that is important is working directly with the patients and with physicians, to understand what kind of interface is most valuable, what kind of information is superfluous, what kind of information is critical, how should that be presented to the individual?"
Doctors and scientists at the Ann and Robert H. Lurie Children's Hospital of Chicago are using his devices to aid preemies and critically ill babies in NICU, replacing the wires and taped on sensors used to monitor vital signs with small, wireless, skin-like patches. The wireless technology allows easier access to holding and caring for the infants.
John Rogers: "We are in the NICU and a regular basis these days, working with about two babies a week, validating the technology, comparing the data streams that we can capture against those that are being recorded with the old technology approach. And it looks very, very promising. Everything works extremely well. I think the parents are happy."
Shirley Ryan AbilityLab scientists and physicians are using Rogers' technology to address challenges around rehabilitation of patients who have suffered from a stroke.
John Rogers: "One type of device that we've designed to integrate with the throat. It actually mounts on a very special part of the body known as the suprasternal notch. It's sort of a soft tissue region just above the collar bone. And, that location was identified to us as important for stroke patients because a device placed at that position can be used to monitor the mechanical acoustic characteristics of speech and swallowing."
The technology for another device he's created, a tiny UV sensor that can be applied to fingernails or on clothing, to detect overexposure to sun. The device is being studied by June K Robinson, MD, a Northwestern Medicine skin cancer expert and the technology has been acquired by L'Oréal for mass production.
John Rogers: "We've tailored an app to extract information (from the UV device), then present it to the user in a way that's most impactful. Dr. Robinson has designed an hour glass to graphically represent the dose that you received up to a particular point and how much additional UV you can you can tolerate before, before it becomes problematic."
Subscribe to Feinberg School of Medicine podcasts on iTunes.
Recorded on June 25, 2018.
This podcast episode is not eligible for AMA PRA Category 1 Credit(s)™.
Erin Spain: This is Breakthroughs, a podcast from Northwestern University Feinberg School of Medicine. I'm Erin Spain, editor of the Breakthroughs newsletter. There are dozens of projects taking place here at Northwestern that involve small electronic devices that can bend, stretch, twist and integrate on and within the human body to both diagnose and treat disease. The devices are created by John Rogers, a pioneer in the field of bio electronics. His primary appointment is in the McCormick School of Engineering and Applied Sciences, and he has an additional appointment here at Feinberg and Neurological Surgery. Thanks for joining me today. John.
John Rogers: Pleasure to be here.
Erin Spain: If someone just googles your name and they look under images, they're going to see some of these devices you've created because they've made some headlines and you have a few of them in front of me here. They are tiny, small patches and temporary tattoos and little tiny pieces, devices that sit on top of fingernails. Tell me about these devices in front of me. What are they made out of? What do they do? So
John Rogers: There's kind of a whole collection of devices here, you know, designed for various different kinds of purposes. All of them integrate with the body in ways that are novel compared to what's possible let's say with a conventional wearable device.
Erin Spain: A Fitbit or Apple watch...
John Rogers: And those, those are great pieces of gadgetry. I mean, you think about though the, the precision of the measurement and the way that they integrate with the body. It's really fundamentally limiting in terms of you know, the kinds of physiological signals that they can detect and sense in a meaningful way. And so an Apple watch or a Fitbit, it's, it's an interesting device and I think it has an important role to play in sort of fitness and wellness. But when it comes to clinical grade measurements of data streams that physicians know how to interpret and analyze and build diagnosis around, they really fall short. And my opinion, and my belief, and I think this is shared by a lot of folks at this point, is that the lack of an intimate interface to the skin is, is what really prevents those classes of devices from measuring things that are commonly quantified in a clinical or laboratory setting because the electronics modules in those kinds of wearables are basically rigid, planar blocks of electronics. And it's great for a lot of things, but it rattles around in a loosely coupled fashion to the wrist or sometimes the chest and the absence of that intimate interface really allows you to make measurements of things like steps or activity level, a rough approximation of heart rate. But it can't allow you to capture electrocardiograms for example. It doesn't allow you to measure, do atrial trigonometry. You can't measure the time dependence of pulsatile blood flow through near surface arteries. You can't measure a electroencephalograms. You, you can't precisely quantify the hydration level of the skin or the thermal character. There's a whole set of things that are done routinely in the clinic that cannot be reproduced with that type of technology platform. And so one theme around the kinds of devices that we've been developing is around new electronic materials, new designs in electronic systems and associated biosensors that allow us to build technologies that are naturally compatible with the skin itself. The kind of vision that we've had in this area and, and sort of the guiding, you know, theme around what we're trying to do is to try to reproduce electronic function in a platform that's reminiscent of a children's temporary tattoo because we feel that, that kind of technology represents the optimal way to integrate devices with the skin in a way that's essentially imperceptible because the technology's adopt the physical characteristics of the skin itself and which provide that continuous intimate interface to the skin such that you can use the skin itself is a window into making all of these sort of clinical grade measurements of health status. And so we have a variety of different platforms that all share that common physical, set of characteristics. They are either electronic in their functionality, we have devices now that our microfluidic in their operations, so we can capture sweat, we can do real time biomarker analysis of sweat, we have devices that can measure ambient conditions, pollution levels, uv exposure levels and so on. And so it's been a very productive direction for us. It's a platform and we take it in different directions. Typically, you know, identifying unmet clinical needs in close collaboration with, with folks at Feinberg. And that's been a powerful a way for us to move forward.
Erin Spain: I know a lot of these devices actually hook up to apps that you can use on your phone.
John Rogers: Yeah. So that's another interesting aspect of this. We're not software engineers, but we have come to appreciate the value of the software component because that's the interface that people are interacting with these devices through. The devices themselves, they don't have switches or displays or there anything else. Everything is happening in a virtual mode through, through an app typically running on a tablet or a smartphone. So, so we've developed internal software expertise in my group. I mean I had to hire a software engineer to do this sort of stuff and we've gotten pretty good at it. And, and I think one thing that, again is important is working directly with the patients, with the physicians, to understand what kind of interface is most valuable, what kind of information is superfluous, what kind of information is critical, how should that be presented to the individual? It's all super important stuff and you know, being embedded in that kind of medical community with the patients and the doctors and us as engineers really allow us to do things pretty quickly.
Erin Spain: Babies in the NICU who are covered in wires. This is another example of how you're using the technology to be less invasive, especially with these very fragile patients. That project. You're doing some trials right now.
John Rogers: Yeah, that's right. So we have a great set of collaborators in neo neonatology and pediatrics, Aaron Hamvas, Debbie Weese-Mayer, and folks in dermatology as well, Amy Paller and Steve Xu, and others. And so, if you go into neonatal intensive care unit, you'll see really a rat's nest of wires and taped on sensors that are being used to monitor vital signs of these premature babies whose health status is very fragile, you have to do that kind of monitoring 24/7 to keep track their health state. And so it's a problem because of the wires themselves can impart forces at the interface between the sensors in the skin. And so the tapes that are needed to keep the sensors adhered and coupled to the skin, have you adhesion strength that's sometimes problematic because repeated application and removal of those tape's can damage the fragile skin of the neonate. The presence of the wires also makes it extremely complicated to even do the most basic manipulation of the baby. You want to turn it over or move it, you want to take it out of the isolet. The mothers want to hold the baby, you got to take all the wires off, put them back on, it's a problem. And then once the wires are there, it really prevents the natural motion of the baby because their muscles are not working very well developed. You got all these mass and sort of physical constraints associated with the wires. It's clearly a non ideal situation. And so we decided, and this was a clinical need that even we understood, you know, before coming here to Northwestern. So about five years ago we decided if we could make these skin-like tattoo based electronic sensor systems work, the first thing that we would want to do is get rid of all the hardwired monitoring technology that's currently used in the NICU. So, so we've been working on that problem for probably four or five years. Over the last year or so, we've been able to engage with all the experts at Lurie Children's and dermatology, neonatology and so on. And we have IRB approval we are in the NICU and a regular basis these days probably, doing two babies a week, validating the technology, comparing the data streams that we can capture against those that are being recorded wit kind of the old technology approach. And it looks very, very promising. I mean everything works extremely well. I think the parents are happy.
Erin Spain: I would say to be able to hold your child without having all the wires in the way it has to be pretty gratifying for them and for you.
John Rogers: Yeah, it's, it's interesting because, you know, you have to consent the parents, they have to understand what you're proposing to evaluate on their baby. Right? So, so you have these conversations and most of the parents of premature babies are pretty stressed out. I mean it's a very stressful and in spite of that, we have had about a 90 percent compliance with our request to do these evaluations because I think even though there's no direct benefit for these parents, they see the value, right, in, in what we're doing. And so they're enthusiastic about helping in the development of the technology, so it's, it's a very satisfying area to work in and I think it really leverages to the maximum extent this kind of skin-like physical form in the devices.
Erin Spain: So one really exciting project you're working on with members of our physical medicine and rehabilitation department, and those are also physicians at the Shirley Ryan Ability Lab is a collaboration where you're working with stroke patients and you've created one of your devices is an actual throat patch in this case. Can you tell me about that?
John Rogers: Addressing challenges around rehabilitation for patients who have suffered from a stroke turns out to have a good alignment with the kind of unique features that we have in our device platform. So we're actually deploying three separate systems on stroke patients today. You referred to one type of device that we've designed to integrate with the throat. It actually mounts on a very special part of the body known as the suprasternal notch. It's sort of a soft tissue region just above the collar bone. And, that location was identified to us as important for stroke patients because a device placed at that position can be used to monitor the mechanical acoustic characteristics of speech and swallowing. And those are two sort of natural body functions that kind of breakdown, you know, in many patients who've suffered from a stroke. The ability to speak in a regular manner with a regular cadence. The regular frequency is impaired as a result of the stroke. It's known as aphasia. And the way that the physical rehabilitation experts at the Ability Lab in the past have sort of monitored talk time, is with a microphone typically on a smartphone, for example, which in principle that works, but you can imagine the kind of confounding signals that, that become problematic just associated with the ambient noise or associated with the voice of a person that you might be having a conversation with. So it became difficult in a practical way to monitor, talk time using that kind of approach. But for these devices and because they're so soft, you can mount them on the neck without any kind of discomfort. We can actually measure the physical vibrational signatures of speech because you're right there near the vocal cords. And so you become completely immune to ambient noise, because you're not measuring sound waves, you're measuring physical vibrations. And so, with extremely high precision and extremely high robustness, we can measure talk time, we can measure talk cadence, we can measure patterns of speech continuously through the day and with the same device, we can measure frequency of swallowing, because swallowing becomes impaired as well. And that's known as dysphagia and I didn't know anything about aphasia or dysphasia, absolutely fantastic. Ans you know, I think the fact that our devices have this skin-like characteristic allow you to contemplate, you know, a mounting positions such as the suprasternal notch. I mean, you couldn't like strap a Fibit to your neck, it's just not gonna work, right? But, but these new kinds of platforms, the new materials that we're using really open up all sorts of possibilities that nobody's really thought about before. And it's really that teaming relationship, that intimate collaboration that makes all this stuff work.
Erin Spain: And talk about personalized medicine for these individuals. You're able to gather so much data on them that didn't, there's no way that could have been gathered before.
John Rogers: Right. And so that, that's the whole point is that I think the model going forward, and this is something that you know, the, the folks that the Ability Lab really, really believe in is that the rehabilitation protocol and the routines will be tailored to the individual in a very precise way. So, by measuring, talk time and swallowing, you can use those parameters as a condition of the status of the patient and you can correlate changes in those measured characteristics to particular features of the rehabilitation process and really refine it with the idea that, that will accelerate recovery and improve outcomes. Now all of this is brand new. I mean, people have not done this in the past and so that's the hypothesis. I believe in it, but, but we'll see how it plays out. I mean, we have devices now on a number of different stroke patients and we're collecting lots of data. One of the additional important aspects of this work is on the data science, like how do you extract detailed information on these measurements? And there we work with with a team of experts in data analytics at the Ability Lab. So 20 person team and they develop all kinds of classifiers and computer algorithms that allow us to take the data streams off the devices, extract meaningful information out of it, and provide that information both to the patient and the rehabilitation specialist that's working with them.
Erin Spain: You are working on a little device that sits on fingernails that helps to measure UV rays, tell me about that.
John Rogers: UV light is one of the most powerful employer, prevalent carcinogens that you come in contact with. And I think skin cancer is the most common cancer that people will encounter. I think that the question is how do you promote safe exposure to the sun and safe practices, right? And in terms of spending time outdoors and an important component of that is creating quantitative awareness around the extent of UV exposure at any given time. And I think one of the problems is people kind of lose track of how much sun they're getting. Right? And there have been wearable devices in the past, sort of your wrist, a wristband or the colors or you know, there was a Microsoft band type of device that had a, a UV sensor embedded in it. June Robinson has had a lot of experience with those kinds of devices. The problem is that people will use them but then the, the consistency around use is quite limited. People will use them for awhile and then they'll end up in the drawer and, and they, they just won't, you know, take, take advantage of that technology. I think part of it has to do with kind of the bulky form factor. Typically a large, larger sort of device something that's going on your wrist. Maybe you don't want something on your wrist if you're engaged in or aquatic activities or something like that. The other problem is that you have to manage the battery, you know, you have to remember to charge or not and then you know that or gets depleted in the middle of the day and you don't want to have to worry about recharging. So we decided, in addition to thinking about electronic devices with these sort of soft, flexible characteristics for integrating on the skin, the other way to think about body integrated device engineering is to make the devices as small as possible. The idea is they get smaller and smaller and lighter in weight. They are less cumbersome and there's less of a hurdle to adoption as a result. And so what we're able to come up with in this particular case in which serves as a centroid of NIH funded studies that we're doing with June Robinson right now is a device platform that's about the size of an M&M candy. I mean it's maybe an eight millimeter diameter, a device. The thicknesses comparable to that of a credit card. The total weight is about 200 milligrams, which is about the weight of the raindrop roughly, you know. So it's small enough, you can put it on a thumbnail or a fingernail, but you could also mount it on a btn btn-primary on your shirt, for example, small clip, you could put it on your sunglasses or clip it on your watch band. I mean there's just a whole variety of different ways that you can deploy this device on on the body because of it's extremely small size and I think that the key feature of the technologies that we figured out how to do continuous digital UV sensing in an accumulation mode, that does not require a battery, that that's the key.
Erin Spain: The key, because there's none of that cumbersome charging as you're talking about.
John Rogers: Yeah. You never have to worry your batteries charged or not. It's always on. It's never off, there no switches so the thing, the device is completely encapsulated in plastic, so it's waterproof and the interface to the phone is is wireless, using the protocols that were originally installed on the phone for wireless payments. So we're using that same kind of wireless capability, which is a present all smartphones these days. And so you can think about the device almost as a being solar powered in a sense. The UV photons themselves are converted to electrons and then those electrons are stored on a capacitor. And then we use the wireless interface to the phone to measure the accumulated voltage on that capacitor. And with the calibration factor, we can convert that voltage into total cumulative dose of UV that that you've received as you're moving around out, outdoors. And so it's a pretty interesting class of device. And, you know, I think through the human subject studies in the field trials that we're doing with June Robinson, we've also tailored the app to extract that information, then present it to the user in a way that's most impactful. She has kind of an hour glass way to graphically represent the dose that you received up to a particular point and how much additional UV you can you can tolerate before, before it becomes problematic. So, it's been a great interaction. And we figured out how to manufacture these devices. So we have a domestic supply chain instead of manufacturing partners, we can build hundreds of these things and deploy them at a meaningful scale so we can get statistically relevant information on how individuals are using these devices and whether, ultimately the most important thing is whether they are changing their behavior, as a result of this increased awareness.
Erin Spain: And I noticed you're kind of onto something with even customizing some of these devices to look like something someone might really want to wear. This could even pass for nail art or something on someone's fingernails. Some of your other devices look like they actually look like temporary tattoos with a heart, with a band around it or even a logo of a company. You found something there that connects with people that they can kind of express themselves are brands can express themselves.
John Rogers: Well, yeah. It's an interesting point. Is a whole other, you know, aspect, a very important aspect of design. You know, it's not just the engineering aspects, but what does it look like from an aesthetic standpoint? What we've found is that in most cases if a device is integrated with the body itself, people are very aware of the appearance and that can be determinative in terms of whether they will be willing to use the device or not. And so we've, we've started to pay attention to that more and more and more. For the fingernail devices, we have a collaboration with L'oreal. They are in a position to launch this technology at a global scale and we're in the middle of a process of technology transfer to allow them to do that. And so they know everything about fashion and style and beauty and we've let them take the lead on adding graphics and colors on top of these fingernail UV dosimeter. In some of the skin interface devices. In some cases we've tried do the best that we can, you know, in terms of our limited but, but non zero sense of aesthetics. In the case of the sweat collecting devices, there's an interesting opportunity there associated with the ability to add colored food dye to the device platforms themselves such as sweat moves up into the device that adopts the color of the dye and depending on the geometry of the channels, you can create almost like a time varying skin tattoo in a sense driven by sweat flow. And so we played around with that a little bit. We tried our best to make something that was visually interesting for the first publication that we had on this technology and ended up on the cover of the scientific journal, Science Translational Medicine. And that was actually my, my first paper as a Northwestern employee. It came out in November 2016, but it captured a lot of public attention in the broader popular press. And one of the places that got interested in. It was a MOMA, the museum of Modern Art in New York City. And the museum curators contacted us about featuring our sweat tattoos in MOMA as part of an exhibit that they were doing on fashion and the history of fashion and the future of fashion. And so it was the coolest thing to have our devices in MOMA for a six month, you know, a feature, a display.
Erin Spain: I'm sure your training as an engineer never prepared you to be a fashion icon.
John Rogers: It is the most amazing thing. So I have all kinds of pictures or a display, a little, you know, tag, there's like John Rogers born 1967, you know, American or whatever. And so there were a 111 items featured in that display and the title of was is fashion modern, something like this. And of the 111, they chose three to be featured in the permanent collection at MOMA and our devices, yeah, so we're permanently MOMA.
Erin Spain: So people want to up close look, they can head to New York.
John Rogers: Yeah. It's there. And one of the interesting questions that they ask as, as part of insertion into the permanent collection was whether any of the colors in our devices would fade over 100 year timescale. I said, I have absolutely no idea. We are not engineering these for, but that's, you know, kind of the timeframe that you want to think about.
Erin Spain: I feel like we're going to be seeing these devices out in the general public soon. It sounds like there's a lot of interests from Gatorade, L'oreal, there's companies that want to get this out to consumers. I think there's an appetite there that consumers want their own data as well.
John Rogers: Yeah. Right. And we're, we're pretty excited about that as well because I think it's kind of commercial pull that can help to drive the technology forward and allow us to add new capabilities and allow us to get even more traction on the clinical side. So, so gatorade is interested the sweat monitoring devices for obvious reasons. They want to create an awareness in their customer base around sweat loss and electrolyte loss as well. So we have the ability to measure chloride concentration in the sweat as it emerges from the skin.
Erin Spain: Ten years from now, do you think everyone's been walking around wearing these devices?
John Rogers: I think there is a very, very high probability that that's going to happen at one level or another. And so, I think these technologies are, are pretty much on rails in terms of where we believe that they're going to go. and so we do a lot of work in ensuring that that translation happens effectively. But then we also have kind of exploratory over the horizon type stuff that we work on as well. A couple of examples would be a biodegradable electronics. So we now have complete sets of materials that allow us to build sophisticated electronic devices that are uniquely characterized by the ability to dissolve in water or bio fluids to biocompatible end products. And so we can imagine now temporary implants that you can insert into the body, they can be designed to provide a diagnostic or therapeutic function timed to a natural body process. After that body process is completed, then the devices, our program to essentially dissolve and disappear. So, the analogy would be to a resorbable suture and you put the suture and you really only needed until the wound is healed and closed, the sutures naturally disappear. Now what we're talking about, our resorbable electronics that support all kinds of sophisticated function in monitoring, electrical stimulation, drug delivery, and so on. And so as one example, we have devices that are configured to implant at sites of a damaged peripheral nerve. They can wirelessly deliver electrical stimulation to the damage site to accelerate the rates of neuro regeneration and improve the efficacy of the healing process over time. Now, in that kind of a circumstance, you don't want the device to be there forever. You just want it to be present while the healing process is ongoing, after the nerve is healed, you don't need the device anymore. You don't want to have to go in and extract it. That would require a secondary surgical operation that could damage the nerve that just healed. So the ideal thing is for that device to be configured to just bio resorb and naturally disappear. So you can almost think about a device like that as an engineered form of a medicine. Because like a drug, it's only present in the body for a finite amount of time, unlike a drug, however, it's not going everywhere in the body, you're locating it at the position where it's function is relevant. And so our hope is that it will open up new avenues for treating disease and accelerating a wound healing, but in a way that minimizes side effects, because the devices are not going randomly everywhere in the body that they're, they're really engineered in a deterministic way, at a specific location. So there's a whole set of devices with this kind of bio resorbable feature that we're working on, not just nerve stimulators, bone stimulators, temporary cardiac pacemakers. We have pressure and temperature sensors that go in the intercranial space during a recovery process after a severe traumatic brain injury. We have programmable drug release vehicles, all of which are bio resorbable in that sense. So, there are couple of other things that we're working on that are kind of out there a little bit. You know, this is not going to be something that's deployed in a year or two, like, you know, we hope our NICU devices will be, but, but we think it could establish a foundation for additional options right in, in treating, treating patients in the future.
Erin Spain: Read more about John Rogers at work and the upcoming summer 2018 issue of Northwestern Medicine Magazine.